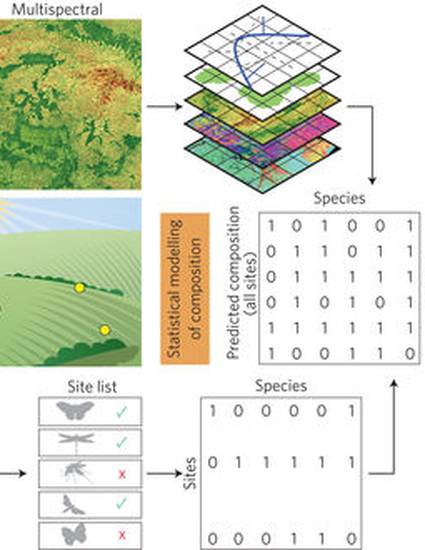
Article
Connecting Earth Observation to High-Throughput Biodiversity Data
Nature Ecology & Evolution
(2017)
Abstract
Understandably, given the fast pace of biodiversity loss, there is much interest in using Earth observation technology to track biodiversity, ecosystem functions and ecosystem services. However, because most biodiversity is invisible to Earth observation, indicators based on Earth observation could be misleading and reduce the effectiveness of nature conservation and even unintentionally decrease conservation effort. We describe an approach that combines automated recording devices, high-throughput DNA sequencing and modern ecological modelling to extract much more of the information available in Earth observation data. This approach is achievable now, offering efficient and near-real-time monitoring of management impacts on biodiversity and its functions and services.
From Google Earth to airborne sensors, the Copernicus Sentinels, and cube satellites, Earth observation is undergoing a rapid expansion in capacity, accessibility, resolution, and signal-to-noise ratio, resulting in a recognized shift in our capability for using remote-sensing technologies to monitor biophysical processes on land and water (1,2,3). These advances are motivating calls to use Earth observation products to manage our natural environment and track progress toward global and national policy targets on biodiversity and ecosystem services (4,5,6). Foremost among these policies are the Strategic Plan for Biodiversity and the Aichi Biodiversity Targets, which were adopted in 2010 by the Parties to the Convention on Biological Diversity (CBD) to “take effective and urgent action to halt the loss of biodiversity in order to ensure that by 2020 ecosystems are resilient and continue to provide essential services…” (7). The United Nations Sustainable Development Goals (8) now include some of the Aichi Targets, and the 2015 Paris Agreement has reiterated the commitments of the UN Framework Convention on Climate Change to reducing emissions from deforestation and forest degradation (REDD+) and to securing non-carbon benefits, which include biodiversity and ecosystem services (9).
However, we have struggled to track and report progress toward the Aichi Targets in a standardized and comprehensive way (10). Although almost two-thirds of the CBD Parties have updated their national biodiversity strategies and action plans to reflect the 2010 revisions, many still do not contain measurable indicators on the state of biodiversity, let alone ecosystem services. This lack of quantification conceals the impacts of policy and management interventions on biodiversity and ecosystem functions and services (11). The difficulty of designing indicators (12,13,14) has prompted an international consortium of biodiversity scientists called GEO BON (Group on Earth Observations’ Biodiversity Observation Network) to propose a framework of Essential Biodiversity Variables (15), with the aim of setting minimum standards of coverage to ensure informativeness and to harmonize disparate local measures so that biodiversity and ecosystem data can be compared over space and time. The Essential Biodiversity Variables thus measure the ‘state of biodiversity’ at multiple levels: genetic composition, species populations, species traits, community composition, ecosystem structure, and ecosystem function15.
Although it was originally envisioned that most of the variables (genetic to community composition) would be scaled up from “intensive in situ measurements” (15) taken on the ground, such measurements are costly and difficult because they are traditionally gathered by visual and aural detection of plants and animals in the wild (preceded by months or years of observer practice) and by mass collection of organisms (followed by months of identification from morphology), so that data collection is slowed by human-caused bottlenecks in sampling and taxonomy (16).
As a result, attention is now being focused on designing ‘Satellite Remote Sensing Essential Biodiversity Variables’ (SRS-EBVs) to enable cost-effective and global-scale monitoring (5,6,12). The problem here is that only a few Earth observation products can be mapped directly to Essential Biodiversity Variables and then to Aichi Targets, because these products primarily measure gross vegetation and landscape metrics, such as land cover and phenology (4). For example, a recent study (12) found only two Earth observation products (net primary productivity and fire incidence) that could serve as Essential Biodiversity Variables for the Sahara, despite this biome's suitability for remote sensing due to its visible biodiversity hotspots, remoteness, and availability of long time series. Many of the Aichi Targets require data with species-level resolution, either because some species are direct policy targets (for example, Target 9: invasive species controlled or eradicated) or because species compositional data define the metric (for example, Target 11: protected areas are ecologically representative and conserved effectively).
Clearly, a radically new approach is required if progress towards the Aichi Targets is to be accelerated, one that is robust, widely affordable, and can record stocks and changes in biodiversity and ecosystem services consistently, continuously, and at high resolution over large geographic scales. Here, we present such an approach in a framework that exploits recent efficiency gains and analytical breakthroughs in sensors, computation, ecology, taxonomy, and genomics (Fig. 1, Box 1).
Keywords
- Biodiversity,
- Conservation biology,
- Decision making,
- Ecosystem services,
- Environmental impact
Disciplines
Publication Date
June 22, 2017
Citation Information
Alex Bush, Rahel Sollmann, Andreas Wilting, Kristine Bohmann, et al.. "Connecting Earth Observation to High-Throughput Biodiversity Data" Nature Ecology & Evolution Vol. 1 (2017) Available at: http://works.bepress.com/john-olson/4/